Ever gazed up at the night sky and wondered about those tiny, man-made stars silently gliding across the darkness? Those are satellites, our eyes and ears in space. But here’s a question that might have tickled your brain: why don’t they just… well, fall down? It’s a fair question! After all, Earth’s gravity is a powerful force, constantly tugging at everything around it.
The truth is, in a way, satellites are falling! Mind-boggling, right? Gravity is indeed pulling them towards Earth every single second. But here’s where the magic of physics (and a whole lot of speed) comes into play. They’re also moving sideways at incredible velocities – we’re talking thousands of miles per hour. This combination creates a fascinating dance: as a satellite falls towards our planet, the Earth’s surface curves away beneath it at the exact same rate. It’s a continuous state of falling, but perpetually missing the ground. Think of it as the universe’s most epic game of tag, where the satellite is always ‘it’ but can never quite catch Earth!
Want a super-quick, animated rundown of this cosmic chase? We’ve got you covered with our YouTube Short. It’s orbital-ly awesome, if we do say so ourselves!
Table of Contents
The Downward Pull: Gravity’s Unseen Hand
Let’s first give credit where it’s due: gravity. This fundamental force, famously conceptualized by Sir Isaac Newton (yes, the apple guy!), is the invisible tether that keeps satellites bound to Earth. Without gravity, a launched satellite would simply yeet itself into the cold, dark expanse of interstellar space, never to be seen again. Every object with mass exerts a gravitational pull on every other object with mass. The more massive the object (like Earth), the stronger its pull.
So, when we send a satellite into space, it immediately comes under Earth’s gravitational influence. This force constantly tries to pull it straight back down. If a satellite were just placed in space without any sideways motion, it would indeed plummet back to Earth, much like an apple falling from a tree.
The Secret Ingredient: Horizontal Velocity – Newton’s Cannonball
Here’s where things get interesting. The key to a stable orbit isn’t about defying gravity, but about having enough horizontal (or tangential) velocity. Newton himself illustrated this with a brilliant thought experiment known as Newton’s Cannonball.
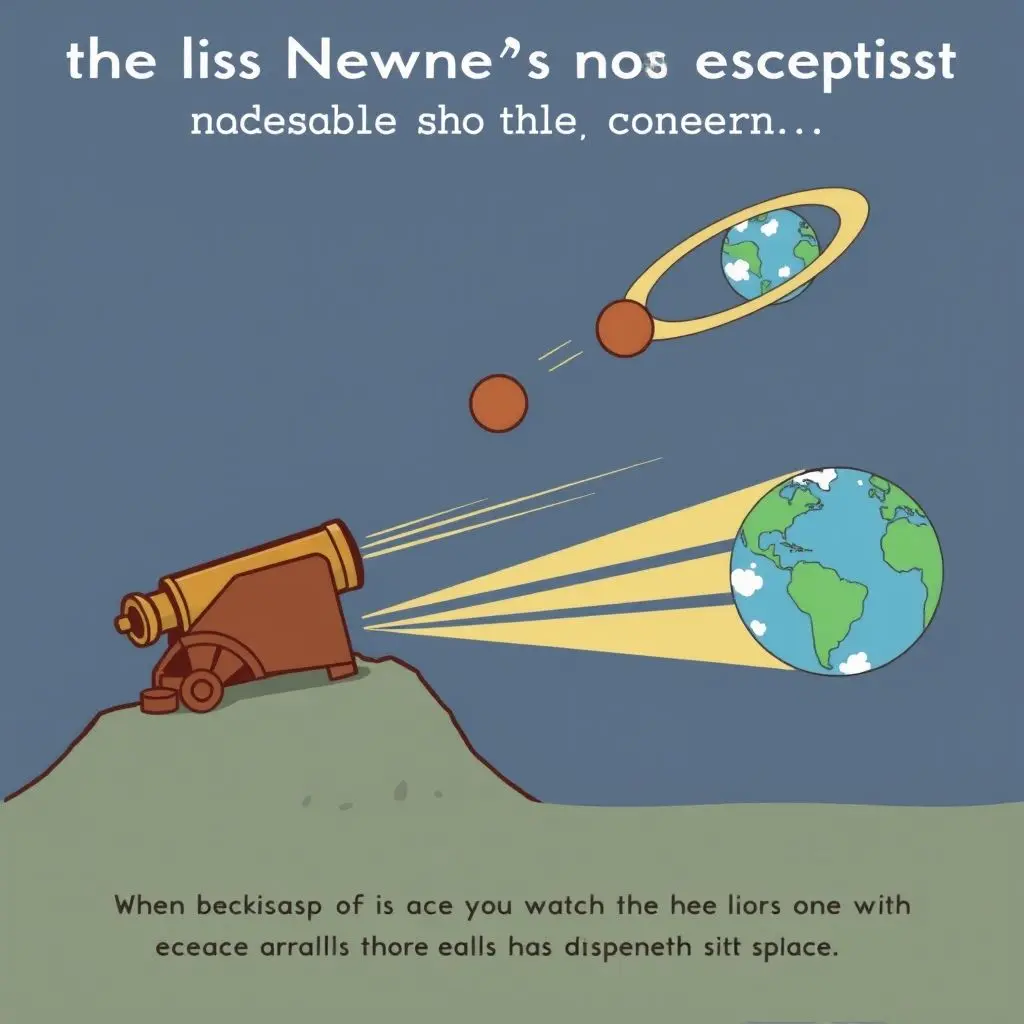
Imagine a cannon perched atop a very, very tall mountain, so high that air resistance is negligible.
- If you fire a cannonball with a small amount of gunpowder (low horizontal velocity), it travels a short distance before gravity pulls it to the ground.
- Add more gunpowder (increase horizontal velocity), and the cannonball travels further before succumbing to gravity.
- Now, what if you could fire the cannonball with such tremendous horizontal speed that as it falls towards Earth, the Earth’s surface curves away beneath it at the same rate?
Bingo! The cannonball would then be in a continuous state of freefall around the Earth. It’s constantly falling, but it’s also moving forward so fast that it keeps missing. This, in essence, is what an orbit is.
The Perpetual Freefall: A Delicate Balance
So, an orbiting satellite is in a constant state of freefall. Astronauts in the International Space Station (ISS) experience weightlessness not because there’s no gravity up there (there’s about 90% of Earth’s surface gravity!), but because they, the station, and everything in it are all falling together around the Earth at the same rate.
This state of orbit is a delicate balance between two main factors:
- The satellite’s forward momentum (inertia): Its tendency to keep moving in a straight line at its current speed.
- Earth’s gravitational pull: The force constantly trying to pull the satellite down towards the center of the Earth.
When these two are perfectly matched for a given altitude, the satellite follows a curved path – its orbit – around the Earth. It’s not using fuel to actively ‘fly’ or ‘hold itself up’; its incredible speed, imparted during launch, is what keeps it from crashing down.
Not All Orbits Are Created Equal: LEO, MEO, and GEO
Satellites orbit at different altitudes, depending on their mission. The altitude determines the satellite’s speed and the time it takes to complete one orbit (its period). Generally, the lower the orbit, the faster the satellite must travel to stay in it, and the quicker it circles the Earth.
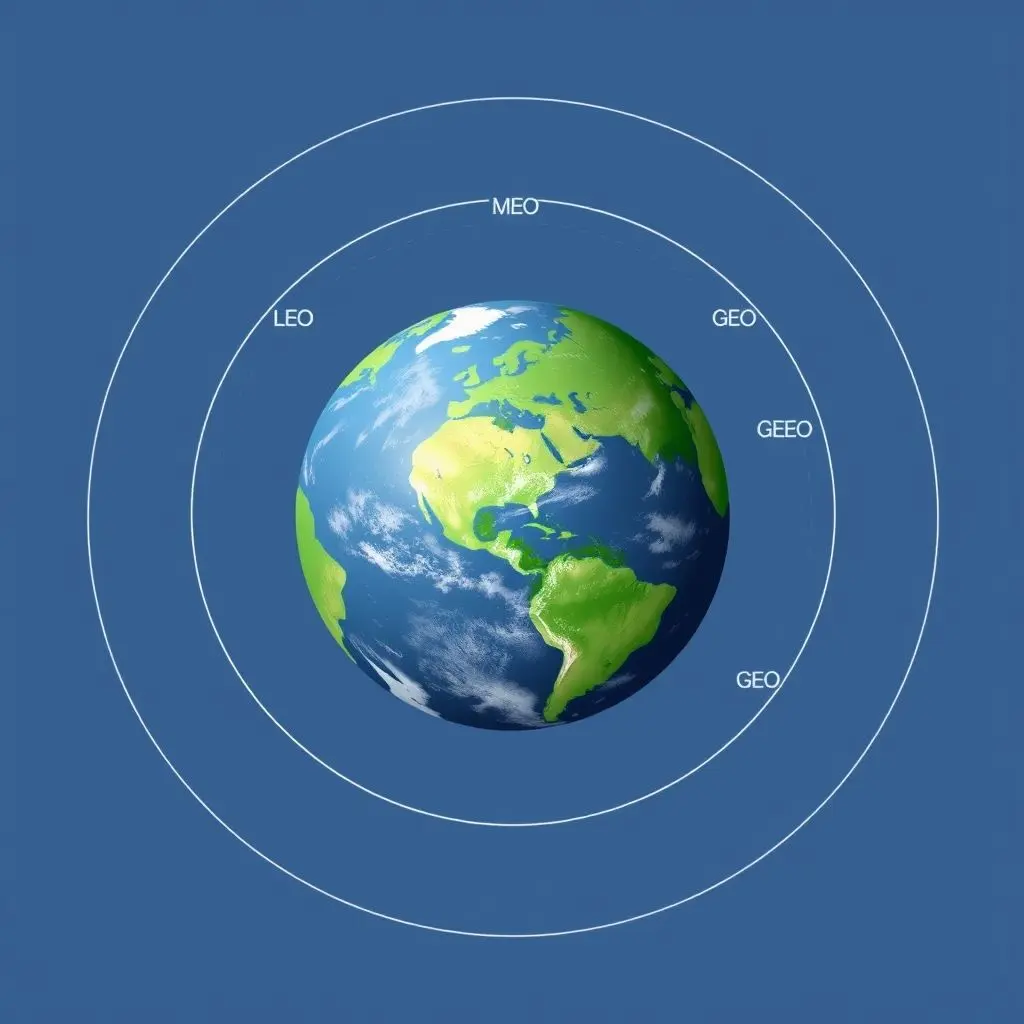
- Low Earth Orbit (LEO): Altitudes from about 160 km to 2,000 km. Satellites here, like the ISS and many Earth observation satellites (think Google Maps imagery), whip around the Earth in about 90 minutes to a few hours. They need to travel at around 17,500 mph (approx. 28,000 km/h)!
- Medium Earth Orbit (MEO): Between LEO and GEO, typically around 20,000 km. This is where GPS satellites reside, taking about 12 hours to orbit.
- Geostationary Orbit (GEO) or Geosynchronous Equatorial Orbit: A special high Earth orbit at exactly 35,786 km (22,236 miles) directly above the Earth’s equator. Satellites in GEO orbit at the same speed as the Earth rotates (about 6,870 mph or 11,050 km/h relative to Earth’s center). This makes them appear stationary from the ground, which is perfect for communications (TV broadcasts) and weather satellites.
Staying on Course: Orbital Adjustments
While an ideal orbit in a perfect vacuum around a perfectly spherical Earth would theoretically last forever without intervention, the reality is a bit more complex. Even in LEO, there’s a tiny amount of atmospheric drag that can slow satellites down over time, causing their orbits to decay. The gravitational pulls from the Moon and Sun can also perturb orbits.
To counteract these effects and to make precise adjustments to their paths or avoid collisions with space debris, satellites are equipped with small thrusters. These thrusters fire periodically for station-keeping maneuvers, nudging the satellite back into its correct orbital slot or altitude. So, while they don’t need continuous propulsion to stay in orbit, they do need occasional boosts.
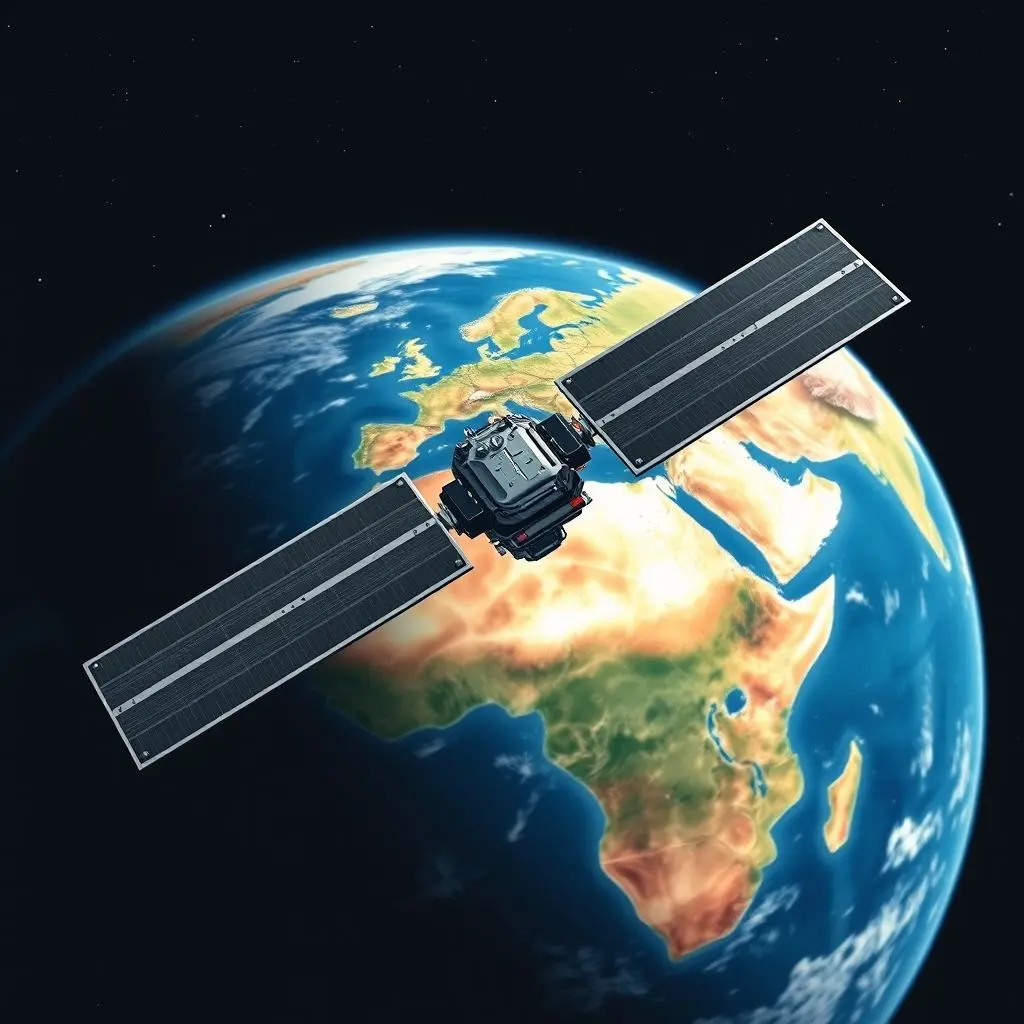
The Cosmic Dance Continues
So, the next time you spot a satellite streaking across the sky, remember the incredible physics at play. It’s not defying gravity; it’s engaged in an elegant, high-speed dance with it – a perpetual fall that never ends, a cosmic game of tag where Earth is always just out of reach. This understanding transforms a simple point of light into a testament to human ingenuity and the fascinating laws that govern our universe.
Frequently Asked Questions (FAQs)
Q1: Do satellites need fuel to stay in orbit?
A: To achieve orbit, yes, rockets need a tremendous amount of fuel. Once in a stable orbit, a satellite primarily relies on its initial velocity and Earth’s gravity. However, most satellites carry some fuel for small thrusters used for station-keeping (making minor adjustments to maintain the correct orbit) and for de-orbiting at the end of their life.
Q2: Why don’t satellites just fly off into space?
A: Earth’s gravity prevents this. If a satellite were moving too fast (achieving what’s called escape velocity – about 25,000 mph or 40,000 km/h near Earth), it could overcome Earth’s gravitational pull and fly off into space. Orbital velocity is a precise balance.
Q3: How fast do satellites travel?
A: It depends on their altitude. Satellites in Low Earth Orbit (LEO) travel at around 17,500 mph (28,000 km/h). Geostationary satellites, much further out, travel at about 6,870 mph (11,050 km/h) relative to Earth’s center to match Earth’s rotation.
Q4: Why don’t satellites crash into each other?
A: Space is incredibly vast, even in common orbital paths. Additionally, space agencies and satellite operators track thousands of objects in orbit and plan maneuvers to avoid collisions. However, the risk of collision with space debris is a growing concern.
Q5: What happens to satellites when they are no longer needed?
A: For satellites in LEO, operators often use the remaining fuel to de-orbit them, causing them to burn up safely in Earth’s atmosphere. Satellites in higher orbits, like GEO, are often moved to a “graveyard orbit” further away from Earth to avoid interfering with operational satellites.